Andrew A.Yang
Corr Instruments, LLC
Utilizing the difference in salt concentration of rivers and seas for green energy production has been the subject of many studies since the 1970s.[1] Pressure-retarded osmosis (PRO) is one of the methods utilized for harvesting this type of energy and relies on the use of ion-exchange membranes.[2] Another method is an electrochemical concentration cell, which relies on differing salt concentrations.[3,4] Solar evaporation has been used to maintain the concentration difference in some concentration cells.[5] However, in all concentration cells, two separate streams or two separate liquid compartments are required, making the concentration cells expensive and bulky. The two separate streams or compartments also require additional energy to operate. The objective of this study is to demonstrate a simple concentration cell that is driven by natural evaporation and has only one liquid compartment for producing sustainable energy.
Experimental
Figure 1 shows the experimental setup for testing the prototype concentration cell design. A porous cup was filled with a dilute KCl solution (0.01 to 1 m) and suspended in ambient air. Two Ag/AgCl electrodes were used, with one being placed inside the porous cup to act as the positive electrode and the other one wrapped on the outside surface of the porous cup to act as the negative electrode.
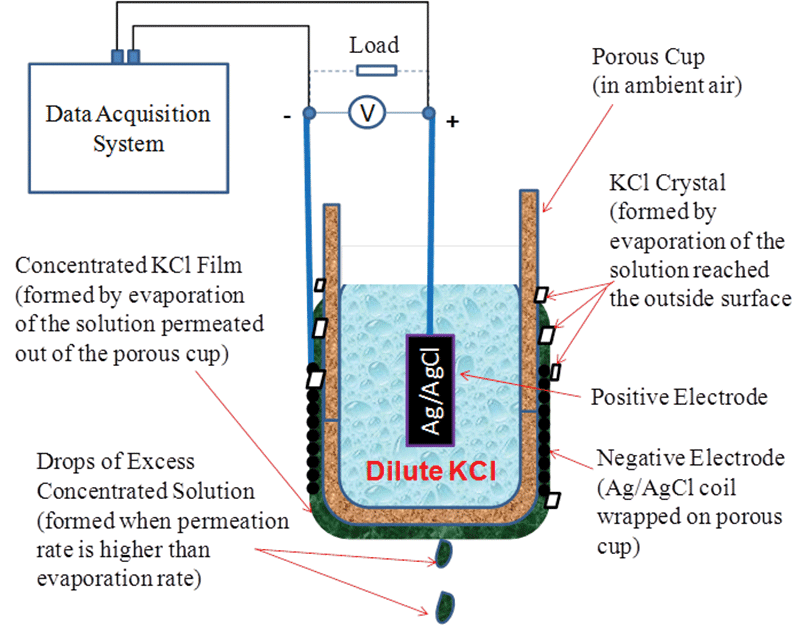
The dilute solution slowly permeated through the porous wall of the cup due to the effects of gravity and formed a thin layer of concentrated liquid film on the outside surface as it evaporated upon contact with the air. Voltage was produced between the positive electrode in the dilute solution and the negative electrode in contact with the concentrated liquid film. The net electrochemical reaction is the consumption of Cl- in the concentrated cell and formation of Cl- in the dilute cell. The open-circuit voltage, Voc, between the two Ag/AgCl electrodes is governed by Nernst’s Equation:
Voc = RT/(nF) ln (ac/ad (eq. 1)
where R is the gas constant (8.314 J/mol), T is the temperature (K), n is the number of the charge involved in the electrochemical reaction, F is Faraday’s constant (96500 C per mol), and ac and ad are the activities of the Cl- in the concentrated cell and in the dilute cell, respectively. In Figure 1, the Voc is the voltage produced between the two electrodes when the load resistor is infinity.
Ideally, the permeation rate is equal to the evaporation rate, and the layer of the liquid film would be saturated by the salt crystal on the outside surface to achieve a maximum concentration difference between the inside and the outside of the cup. When the permeation rate was higher than the evaporation rate, excess liquid drops would fall off the porous cup and the liquid film would be less concentrated; when the permeation rate was lower than the evaporation rate, excess KCl crystals would form and the liquid film would be dried out.
The two electrodes were made of 0.1-mm-diameter silver wire loops with a total surface area of about 10 cm2. The positive electrode was initially chlorodized in a 1-m KCl solution by electrolysis, with a DC power supply. Electrolysis was conducted at 1.6 V until the electrode was covered completely by a black-colored AgCl film.
Figure 2 shows the prototype of the concentration cell. Both a liquid film and KCl crystals were clearly visible on the outside surface of the porous cup, indicating that the KCl in the liquid film was near saturation. A silicone tube was attached to the top of the porous cup and used as a reservoir so that more liquid could be stored to make the cell operate longer without a continuous feed. The silicone tube was also used to increase the liquid level so that the permeation rate of the liquid from inside to the outside was enhanced by gravity.
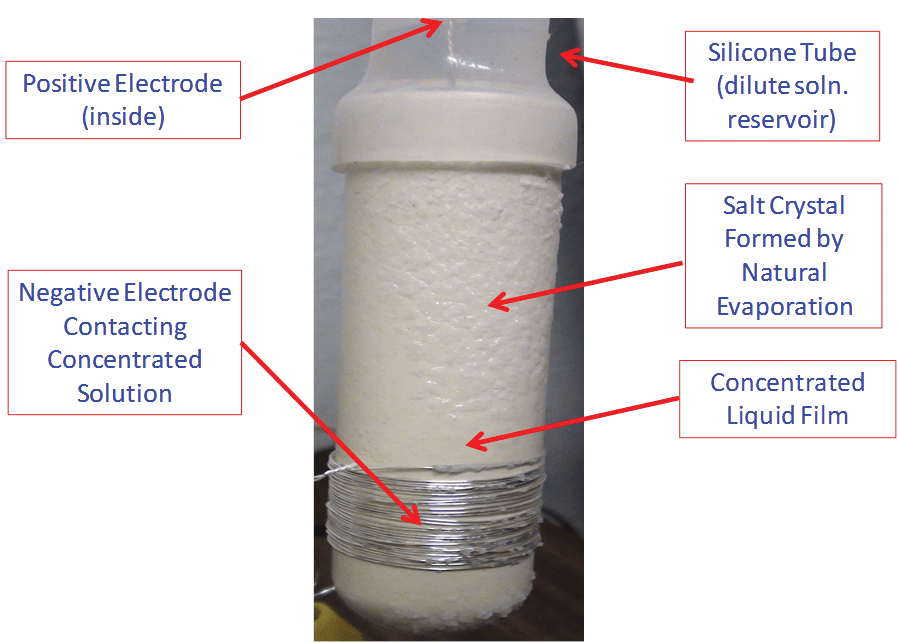
Figure 3 shows a two-compartment concentration cell arranged in a conventional configuration for comparison with the prototype. The two chloride electrodes were used to measure the chloride concentrations in the two compartments. The signals from the two–compartment conventional cell, including the signals from the two chloride electrodes, were measured by the same data acquisition system as shown in Figure 1. In Figure 3, the concentrated solution was placed inside the small porous cup (holding 7 to 10 mL solution) and the dilute solution was placed in the large cup (holding 100 mL solution) to slow down the decreasing rate of the ratio of the chloride concentrations in the two compartments caused by diffusion.
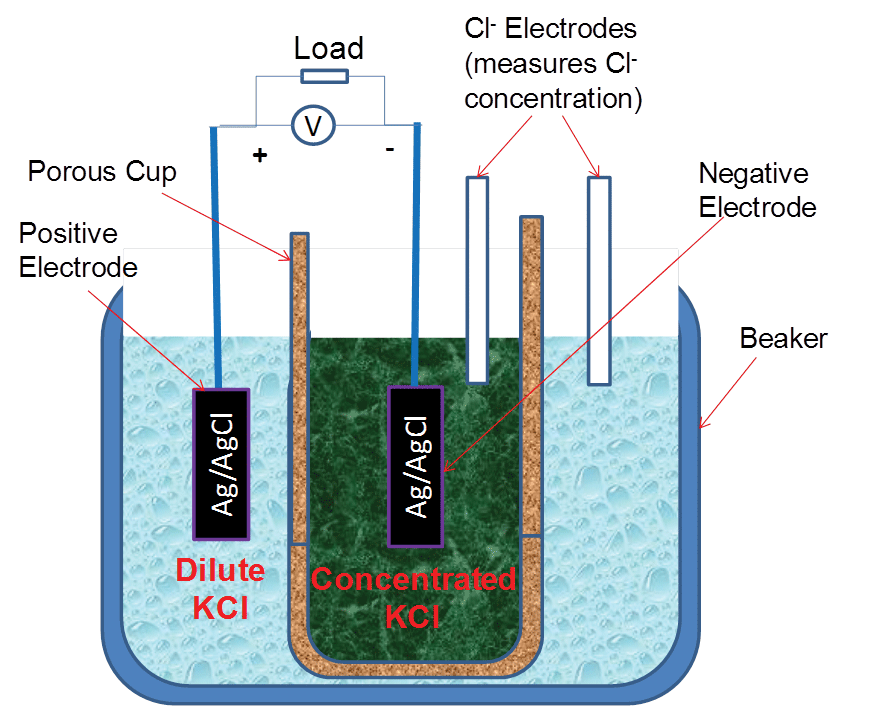
Results
Figure 4 shows typical power density and cell voltage measured from the prototype single-compartment concentration cell (Figure 1) filled with a 0.1-m KCl solution and connected to a 1,000 ohm resistor as a function of time. The data from the two-compartment conventional cell (Figure 3) filled with similar solutions were also presented in Figure 4 for comparison. After the first 80 minutes, the power density and voltage of the single-compartment cell were higher than those of the two-compartment conventional cell. It is surprising to note that near the end of the test (the 1,400th minute), the power density of the single-compartment cell was 400 percent higher than that of the two-compartment conventional cell.
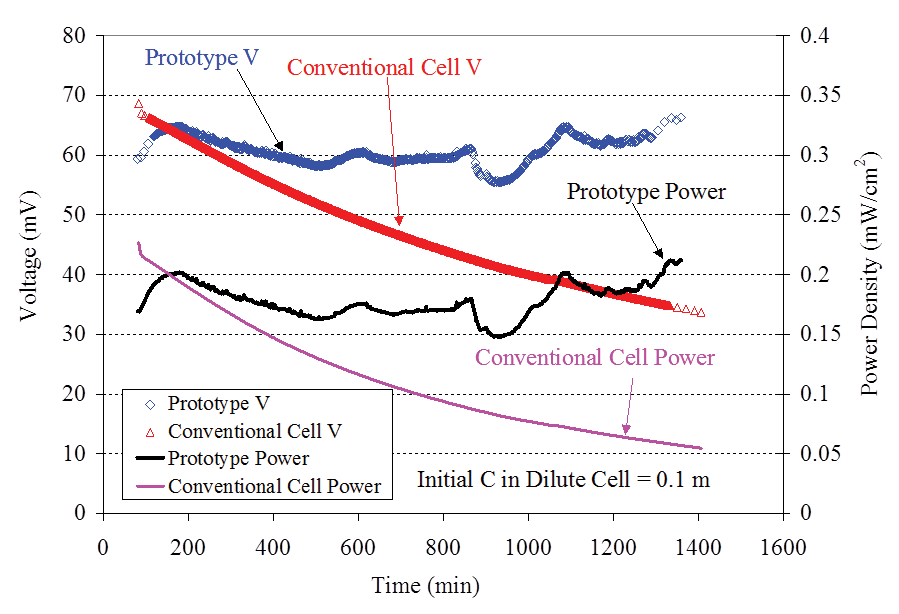
The surface area used to calculate the power density for Figure 4 was the whole outside surface area of the porous cup (20.8 cm2) that was the same as the value used for the two-compartment cell. It is interesting to note that the power density from the single-compartment cell did not decrease with time, which is in sharp contrast with the data from the two compartment cell. The minor fluctuation of the power density from the single-compartment cell was probably due to the fact that the humidity of the room changed, which affected the evaporation rate and hence the concentration of chloride in the liquid film formed on the outside surface of the porous cup.
The data from the chloride electrodes showed that the voltage decrease with time from the two-compartment cell was caused by the rapid diffusion of chloride ions from the concentrated compartment to the dilute compartment. Near the end of testing, the ratio of the concentrations in the two compartments changed from the initial value of 45 to approximately 3.5.
Like thermoelectric power devices, an electrochemical concentration cell has very low voltage (20 to 200 mV) and therefore cannot be used to power commonly used electrical devices. Technologies for converting low-voltage (20 mV) energy to higher-voltage (2 to 5 V) energy are commercially available. In this study, a low-cost voltage step-up converter chip was successfully used to convert the low-voltage (20 to 100 mV) energy generated by the single-compartment concentration cell to 2.3-V energy. The converted energy was able to power an LED (light-emitting diode) for a short duration.
Discussions
In the novel single-compartment prototype, the concentration of chloride in the thin liquid film attached to the outside wall surface of the porous cup was maintained to about saturated value by permeation caused by gravity force and by natural evaporation. Yet, the continuous flow of the liquid from the inside to the outside through the porous wall prevented the diffusion of the chloride ion from the concentrated liquid film on the outside wall to the dilute solution inside the porous cup. This phenomenon was supported by the results from both the power output measurements, and the chloride concentration measurements.
The novel single-compartment concentration cell was much simpler than the two–compartment concentration cell. It eliminated the second compartment in a conventional two-compartment concentration cell. The single-compartment cell also significantly reduced the size of a conventional two-compartment cell, making the dry form of the cell easier to carry and more suitable for use as a mobile device. The single-compartment concentration cell also eliminated the need for energy to maintain the liquid flow in the second compartment (for example by pumping).
Because the concentration difference between the liquids for the positive and the negative electrodes is driven by gravity and natural evaporation, this novel cell operates both indoors and outdoors as long the surrounding air is not saturated by moisture.
Conclusion
The concept of a novel electrochemical concentration cell was developed and a prototype of the cell for power generation was built and tested in parallel with a two-compartment conventional cell. This prototype only had a single compartment for the positive electrode, and the liquid in contact with the negative electrode was a thin film. Cell voltage was generated by the environmentally friendly chloride concentration difference that was continuously maintained by gravity and natural evaporation. This single-compartment cell operated both indoors and outdoors as long the surrounding air was not saturated by moisture. The single–compartment cell eliminated the problems experienced with two-compartment cells. The prototype cell also eliminated the need for energy to pump the liquid flow in the second compartment of a conventional two-compartment cell. As a result, the single-compartment cell also reduced the size of a conventional two-compartment cell, making it portable and more suitable for battery usage.
The test data from the power output measurements demonstrated that the novel prototype outperformed the conventional concentration cell. Off-the-shelf, low-cost chips were available for converting the low-voltage energy from the concentration cells to higher-voltage energy (2 to 5 V) and were successfully used in this study.
References
1. R.I. MacDonald, “Energy Generation and Storage using Evaporated Brines,” 2009 IEEE Electrical Power and Energy Conference (EPEC), Oct 22-23, 2009, (Piscataway, NJ: IEEE International).
2. A. Achilli, A. E. Childress, “Pressure Retarded Osmosis: From the Vision of Sidney Loeb to the First Prototype Installation — Review,” Desalination, 261(3), 205, 2010.
3. R. B. Sadeghian, O. Pantchenko, D. Tate, and A. Shakouri, “Miniaturized Concentration Cells for Small-Scale Energy Harvesting Based on Reverse Electrodialysis,” Appl. Phys. Lett. 99, 173702, 2011.
4. G. Lagger, H. Jensen, J. Josserand, H. Girault, “Hydro-Voltaic Cells, Part I. Concentration Cells,” Journal of Electroanalytical Chemistry, 545, 1, 2003.
5. M. A. Bobrik and E. Austreng “Solar Driven Concentration Cell,” United States Patent Application # 0060204838 (Publication Number: 20060204838), 2006.
For more information on Corr Instruments, please visit www.corrinstruments.com.