By Kent Griffith
December 6, 2022 | Nickel-rich layered oxides with at least 80% nickel—moving rapidly toward 90% nickel—provide the highest energy density of commercialized cathode materials. Examples include NMC811 (LiNi0.8Mn0.1Co0.1O2), NCA (LiNi0.80Co0.15Al0.05O2), and variations thereof. Increasing the nickel content leads to higher accessible charge storage capacity, which translates to longer driving range for electric vehicles, but it also comes with stability challenges during material production and battery operation. Increasing the nickel content also trends with lower cobalt use, thus lowering supply chain challenges and cost variability. Many strategies have emerged to stabilize nickel-rich cathodes such as doping with various cations and/or coating the particle surfaces. In most cases, these strategies do not fully suppress the volume expansion and contraction associated with cycling the cathode in a lithium-ion battery, but they do lessen the detrimental effects such as particle cracking and interactions between the cathode surface and the reactive electrolyte solution and can enable long-term cycling with good capacity retention.
A variation on the doping strategy has been developed by researchers at Zhejiang University in China and Argonne National Laboratory in Illinois. Rather than add dopants randomly into the atomic structure of the layered cathode, they formed a secondary phase of La4LiMO8 (M = Ni, Co, Mn) as an intergrowth with the cathode crystal structure. In doing so, they were able to suppress the change in volume upon battery cycling and achieve a longer cycle life than the undoped material. Battery researchers can use beams of light coming off of particle accelerators to take detailed images and extract chemical and mechanical information about electrode materials. The researchers here did just that to identify mechanisms underlying their battery cycling results. Extensive characterization of the modified NMC811 showed that it possesses lower chemical heterogeneity and suppresses particle cracking or void formation after cycling when compared to the unmodified baseline cathode. The work was published this month in the journal Nature.
Regarding the stabilization approach, about 1% of the La4LiMO8 (M = Ni, Co, Mn) phase is incorporated into NMC811 by adding lanthanum nitrate to the NMC hydroxide and lithium hydroxide precursor particles before firing. This strategy is impressive in its simplicity and efficacy. Although lanthanum falls in the ‘rare-earth’ section of the periodic table, it is not a particularly rare element. According to the U.S. Geological Society, the cost of lanthanum has been stable at $2,000/metric ton for the past five years. It is primarily mined and processed in China.
The secondary phase in this work is referred to as a perovskite, which denotes a crystal with a specific arrangement of atoms, while La4LiMO8 may be more precisely called a Ruddlesden–Popper phase (Figure 1), a layered derivative of the perovskite structure with a slightly different atomic arrangement. According to the perovskite alignment system chart by Robert Palgrave of University College London, calling La4LiMO8 a perovskite falls into the “Composition Neutral / Structure Rebel” category.
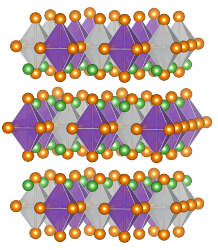
Eungje Lee, a Materials Scientist from Argonne National Laboratory who was not involved in this work, commented (personally, not representing the views or opinions of Argonne National Laboratory): “The concept of having a structurally incorporated secondary phase as a stabilizer to layered LiMO2 is a promising strategy for high-energy, high-stability cathodes. The Argonne cathode group has been leading this strategy for the past decades by developing several new ‘composite’ materials, such as layered–layered (Li2MnO3–LiMO2) and layered–spinel (Li2MnO3–LiMO2–LiM2O4) cathodes. In those materials, Li2MnO3 with a layered structure or LiM2O4 with a spinel structure was incorporated into the parent LiMO2 layered structure to impose additional stability.”
Lee went on to say, “Building on the previous work, our (current and former) Argonne colleagues proposed a new cathode design concept that employs a stabilizer phase. It is interesting that the perovskite phase is introduced as a ‘nano-rivet’ specifically to hold up the volume expansion of the layered cathode during charging, which is a chemo-mechanical source of performance degradation. If such a ‘strain-retardant’ design concept really works as the authors claimed in the paper, it could have significant implications not only for advanced LIBs but also for solid-state batteries, for which high external pressure is required in order to override the cyclic volume change of cathodes and associated disintegration at the cathode/solid-electrolyte interface during operation.”
Alex Rettie, a Lecturer in Electrochemical Energy Conversion and Storage at University College London who was not involved in this work added that, “These are exciting results—the modification to typical material synthesis is minimal and should be compatible with large-scale manufacturing. Impressive electrochemical stability is demonstrated but mostly in small coin-cell format, so it will be important to determine whether this extends to larger, commercially relevant cell formats.”
There are a number of future directions that emerge from this paper. For example, commercial nickel-rich cathodes are typically doped and/or coated, so it will be interesting to see how the perovskite stabilization strategy compares to traditional doping and coating reagents, or whether multiple stabilization methods can be combined with improved results. As allued to by Alex Rettie, long-term stability can often only be detected in commercial-style full-cell formats that come later in the development process after scaling up a new material. In order to improve the energy density, it will also be valuable to increase the active material content and tune the ratio of the secondary phase to boost the accessible capacity. As with any dopant or a coating phase, there is a sweet spot in additive content: too little and you don’t get the stabilizing benefit, too much and you suppress the ability of lithium to travel through the cathode or coating layer.
The formation of La4LiMO8 looks promising under 1C/1C rate testing, which is a realistic charge rate for EVs, but longer-term future studies should explore alternate rates that probe slower phenomena and calendar aging as well as higher currents that simulate acceleration and extreme fast charging. Overall, perovskite stabilization provides another tool in the race toward increasing the energy density of battery cells with nickel-rich cathodes for electric transportation applications.