Contributed Commentary by Lingxi Kong and Michael Pecht
August 24, 2020 | Lithium-ion batteries are the most popular type of portable rechargeable batteries today and are used in many electronic devices. Their proliferation can be credited to their higher energy density, power density, and lack of memory effect. Other types of rechargeable batteries are unable to offer these advantages. Thus, the application of Li-ion batteries has been expanding since the 1990s when they were first commercialized. A Li-ion battery usually contains an anode made of graphite and a cathode made of lithium transition metal oxide. Under normal operation conditions, the lithium ions transfer between two electrodes for energy storage and release, giving the name Li-ion battery to this type of rechargeable battery. However, numerous Li-ion battery safety incidents have been reported, raising public concerns.
Among the reported incidents, internal short circuits caused by lithium dendrite formation were one of the reasons for battery failure. A Li-ion battery operating under abnormal conditions, such as overcharging or lower temperature charging, can lead to a harmful phenomenon called lithium dendrite growth or lithium plating. Lithium dendrites are metallic microstructures that form on the negative electrode during the charging process. Lithium dendrites are formed when extra lithium ions accumulate on the anode surface and cannot be absorbed into the anode in time. They can cause short circuits and lead to catastrophic failures and even fires. Several Samsung Galaxy Note 7 batteries caught on fire in 2016, and the investigation revealed the mechanism that lithium dendrites caused an internal short circuit. Capacity fade is another potential hazard of lithium dendrite growth. The lithium dendrite reacts with the electrolyte, causing it to decompose and triggering the loss of active lithium inside the battery. The capacity loss is an accumulating effect along with the gradual lithium dendrite growth.
Understanding the growth mechanism of lithium dendrites is beneficial for improving battery safety. However, lithium dendrite growth is difficult to observe because the typical Li-ion battery is not transparent. At the Center for Life Cycle Engineering (CALCE) at the University of Maryland, we have created a transparent optical cell to observe the lithium dendrite growth process and address safety concerns. A symmetrical lithium cell, in which both the positive and negative electrodes were made of lithium metal, was charged at a constant current to determine the dendrite growth rate.
Lithium dendrite growth is influenced by multiple parameters, including current density, temperature, electrolyte, and electrolyte convection. These factors determine the electrolyte dynamics. In the current stage, a constant current was applied until a lithium dendrite caused an internal short circuit. The relationship between the applied current density and the dendrite growth rate was the research focus in this step of the study. An in situ observation method was used to detect dendrite formation at various current densities.
The dendrite morphology under various current densities can be seen in Fig. 1. The current densities changed from 10 to 110 mA/cm2. The time noted in each image indicates when internal short-circuiting occurred after the start of the test. In these tests, the lithium dendrite grew from the negative electrode (right) toward the positive electrode (left). While increasing the current density, the time to short circuits decreases, which means the lithium dendrite growth rate increased at increased current density. Besides, the morphology of the lithium dendrite changed. At higher current densities, the protrusion portion (indicated by the red arrows) formed on the edge of the negative electrode.
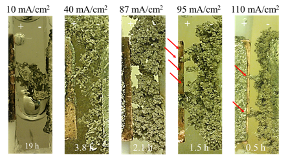
In Figure 2, a typical current and voltage profile is observed during the lithium dendrite growth test. This profile shows the current and voltage from the test under 110 mA/cm2, corresponding to the fifth sample in Fig. 1. In this example, the current was controlled and showed a constant value. The voltage kept almost stable around 0.7 V until a sudden drop at about 0.5 h, which indicates the lithium dendrite caused an internal short circuit. The average dendrite growth rate increased along with increasing current density, which can influence the dendrite morphology. When the current density increased above 87 mA/cm2, the dendrite morphology changed from flat mossy to sharp needle-like in the growth process. Observing lithium dendrite growth and the factors that influence growth helps to mitigate the issues surrounding failures and incidents in consumer technology. The dendrite growth rate captured at the various current densities can be used to estimate the length of the dendrite and provide early warning to short circuits.
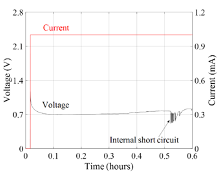
Lingxi Kong and Michael Pecht are researchers at Center for Advanced Life Cycle Engineering (CALCE) Battery Research Group at the University of Maryland. The group can be reached through Michael Pecht, Director of CALCE, at pecht@umd.edu